Effect of surface hardening by induction heating
Views Send Enquiry
Surface hardening of carbon steel by high frequency induction heating (HFIH) produces a considerable increase in its resistance to stress corrosion [1, 2]. The magnitude of this increase depends on the depth of surface hardening, on the strength of the specimen core, and on other factors whose influence was studied in this investigation.
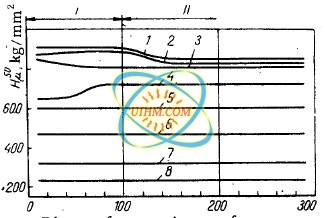
effect of surface hardening
The surface hardening of steel 40Kh specimens was done
by heating them continuously and progressively in a single-coil
inductor of a HF induction set LGPZ-60 (frequency = 200-300 kc,
power rating = 60 KW). The initial structural state of the specimens
varied: some had a pearlito-ferritic structure (produced by
annealing) while some consisted of martensite, troostite or sotbite
(produced by quenching the specimens from 880~ and tempering
them for 2 hr at 180, 850, or 800~ respectively). The
depth of the surface-hardened layer was 0.8, 1.8 mm in martensitic
specimens, 1.2 and 1.8 mm in specimens consisting of
troostite, and 1.4 and 1.8 mm in the case of specimens with a
sorbitic structure. The depth of surface hardening was uniform
in both the radial and axial directions. After the surface hardening,
the specimens were tempered (2 hr at 180~ and ground to
a Class 8 surface finish. The hardness of surface-hardened layer
was HRC = 55-57; the variation in the microhardness with the
distance from the specimen surface is shown in Fig. 1.
Stress-corrosion tests were carried out on cylindrical specimens
(20 mm diam. ) stressed in tension in a 20% I12SO4 solution
at room temperature.
The results showed that the stress-corrosion resistance of
steel with tile initial pearlito-ferritic structure is substantially higher after surface hardening than after ordinary quenchhardening
(Fig. 2, curves 1,4, 5, G).
Increasing the surface-hardening depth from 0.8 to 2. ,5 mm produces a dight increase in the conditional static
corrosion-fatigue limit (stress-to-rapture). The optimum surface-hardening depth for steel with an initial pearlito-ferritic structure is 1.8 mm. A larger increase in the conditional static corrosion-fatigue limit is produced by surface hardening of specimens with a harder (in comparison with pearlito-ferritic) core. And so, surface hardening by HFIH produced a 2.5-2.8 increase in the conditional static corrosion-fatigue limit of specimens which initially had a martensitic, troostitic or sorbitic structure (see Fig. 2), the optimum surface-hardening depth being 0.6, 1.2 and 1.4 mm, respectively.
Although the stress-corrosion resistance of steel after surface hardening is usually higher than after ordinary quenchhardening, the latter treatment followed by tempering at 550 ~ C ensures a much higher resistance to stress corrosion
(Fig..q). Consequently, the surface hardening by tlFItt may be recommended as a means of improving the stress-corrosion
resistance of parts working under conditions that require a high surface hardness.
Data plotted for specimens with different structures surface-hardened to the same depth (Fig. 3, curve 2) or to
tile optimum depth for a given structure (Fig. 3, curve shows that in both cases the maximum value of the conditional
static corrosion-fatigue limit after surface hardening by ttFItt was recorded for steel with a troostitic structure and the
lowest for specimens with a pearlito-ferritic structure.
The differences in the stress-corrosion resistance of steel given a surface-hardening treatment are associated with differences in the structure of the material and in the magnitude of residual stresses produced in the metal surface layers
(Fig. 4)~ Surface hardening by HFIH of specimens with an initial pearlito-ferritie, troostitic or martensitic structure produced surface layers consisting of two zones (I, II) possessing different microhardness (Fig. 1) and structure.
Fig. 2. Stress-corrosion curves of steel 40Kh specimens after the following
heat treatments: 1, 2, 18) ordinary quench-hardening followed by tempering
at 180, 850, and 550~ respectively; 3,4) surface hardening of steel with
a pearlito-ferritic structure by HFIH to a depth of 0.8 ram, curves 8 and 4
relating to specimens with one and several surface cracks, respectively; 5,
6) surface hardening of steel with a pearlito-ferritic structure by HFIH to a
depth of 2.5 and 1.8 mm, respectively; 7, 10) surface hardening of steel
with a sorbitic structure to a depth of 1.8 and 1.4 mm, respectively; 8, 11)
surface hardening of steel with a martensitic structure to a depth of 1.8 and
0.6 mm, respectively; 9, 12) surface hardening of steel with a troostitic
structure to a depth of 1.8 and 1.2 mm, respectively.
The microstructure of the surface layer of steel with an initial pearlito-ferritic structure is characterized after surface
hardening by a highly dispersed martensitic structure and a high microhardness (Fig. 1, curves 1, 5); a certain reduction
in microhardness observed in zone II is attributable to a larger grain size.
In the case of specimens with an initial troostitic structure, surface hardening led to the formation of surface layers
consisting of acicular martensite with an increased troostite content and having, as a result, a slightly lower microhardness
(Fig. 1, curve 4, zone I); this zone changes gradually to finely dispersed martensite with a higher microhardness
(Fig. 1, curve 4, zone II).
Surface hardening of specimens with an initial martensitic structure produces surface layers which consist of finely
dispersed martensite changing to a more coarsely-crystalline martensite with small troostite regions; the maximum microhardness
is observed in zone I (Fig. 1, curve 2).
*The determination of residual axial stresses was done by measuring the strain of specimens from which successive
surface layers were removed by dissolution [3].
Fig. 4. Distribution of residual stresses after surface hardening by HFIH of
steel specimens with a pearlito-ferritie structure to a depth of 0.8 and 1.8
mm (curves 1 and 4, respectively), with a sorbitic structure to a depth of
l. 4 mm (curve 2), and with a martensitic structure to a depth of 0.6 mm
(curve 8).
And so, rapid HFIH during surface hardening of steel specimens with various initial structures leads in every case
to a refinement of the crystal structure and to an increase in microhardness. As a result, residual compressive stresses
are produced in metal surface layers; their extent and distribution depend on the depth of surface hardening, heating and cooling rates, changes in the specific metal volumes and other factors.
Fig. 8. The appearance of fracture surfaces of surface-hardened specmens
of steel 40Kh with the following initial structures: a, b) pearlitoferritic;
c) troostitic; d) martensitic.
When surface-hardened specimens of steel with an initial pearlito-ferritic structure are stress-corrosion tested,
numerous closely situated cracks are formed on their surface; due to the self-relieving effect [5] these cracks produce
a smaller reduction in the conditional static corrosion-fatigue limit than that produced by a single crack* (Fig. 2,
curves 8, 4).
The fracture surface of these specimens is clearly divided into two zones: a zone of the propagation of a corrosion
crack through the surface-hardened layer, and the zone of ultimate fracture in the specimen core (Fig. 8a). In isolated
cases a brittle fracture took place as a result of simultaneous propagation of several cracks, as a result of which a steplike
fracture surface was produced (Fig. 5b).
In the case of surface-hardened specimens with initial sorbitic, troostitic (Fig. 5c) and martensitie (Fig. 8d) structures,
the fracture took place along the path of the first crack formed; metallographic examination revealed no other
cracks in specimens of this kind.
*The formation of a single crack was ensured by painting the specimen gauge portion with a bakelite varnish and
then making a circular incision in the varnish coating to expose a very narrow band of specimen surface.
REFERENCES
1. V.T. Stepurenko, Investigation of the Corrosion and Stress-Corrosion Resistance of Steel St. 45 [in Russian],
Izd. AN USSR, L’vov, 1958.
2. I.I. Vasilenko et al., FKhMM [Soviet Materials Science], no 2, 227, 1966.
3. C.V. Karpenko and B.F. Ryabov, et aL, FKhMM [Soviet Materials Science], no I, 1966.
4. G.V. Karpenko, Strength of Steel in Corrosive Media [in Russian], Mashgiz, 1963.
20 September 1967 Institute of Physics and Mechanics, AS UkrSSR, L’vov
Related Content
induction brazing SS steel by handheld induction coil
induction melting silicon and steel
magnesium oxide acidic furnace for induction melting glass
induction preheating gas pipeline by full air cooled clamp induction coil and DSP induction heater
induction melting with Titanium alloy pot by customized induction coil
induction melting aluminium for casting wire wheel
induction heating steel plate by pancake induction coil
induction heating steel knife
Hot
induction hardening surface of steel plate
induction hardening gear surface
induction hardening wheel gears
induction hardening TCT knife (tungsten carbide tool)
induction hardening knife edge (sickle blade)
induction hardening inner surface of steel pipe
induction hardening inner surface of steel ring by UHF induction heater
induction hardening inner surface of gear
Newest Comment
No Comment
Post Comment